The Pesticides Used in Farming Are Continually Re reviewed by the to Ensure Their Safety
Contents
- 1.
Introduction ................................................................2
- 2.
Crop losses to pest ......................................................5
- 3.
Estimates of pesticide-related productivity ...................9
- 4.
Costs and benefits of pesticide use ...........................15
- 5.
Biopesticides and integrated pest management ..........20
- 6.
Challenges of the global pesticide market ..................25
- 7.
Conclusions ..............................................................28
Introduction
The combined effect of the Green Revolution has allowed world food production to double in the past 50 years. From 1960 to present, the human population has more than doubled to reach seven billion people. In 2050, the population is projected to increase by 30 % to about 9.2 billion (Fig. 1). Due to increasing global population and changing diets in developing countries towards meat and milk products, demand for food production is projected to increase by 70 % (FAO 2009).
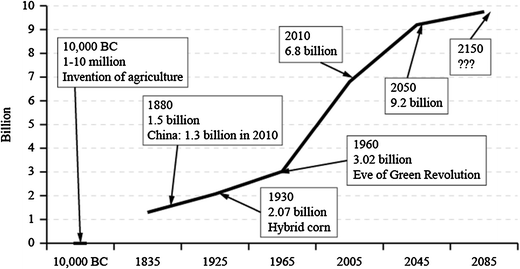
World population growth. From 1960 to present, the human population has more than doubled to reach 7 billion people and in 2050, the population is projected to increase by 30 % to about 9.2 billion. Source: FAO (2009)
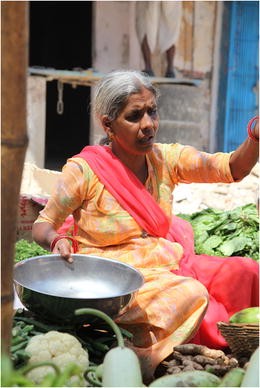
Food market in Udaipur, Rajasthan, India. Copyright: Rémi LE BASTARD—INRA, 2012
Globally, an average of 35 % of potential crop yield is lost to pre-harvest pests (Oerke 2005). In addition to the pre-harvest losses, food chain losses are also relatively high (IWMI 2007). At the same time, agriculture has to meet at a global level a rising demand for food, feed, fibre, biofuel and other bio-based commodities. The provision of additional agricultural land is limited, as agricultural expansion would have to happen mostly at the expense of forests and the natural habitats of wildlife, wild relatives of crops and natural enemies of crop pests. Given these limitations, sustainable production and increasing productivity on existing land is by far the better choice. Part of the key is also to avoid waste along the whole length of the food chain. The increase in production will occur at the same time as the climate is changing and becoming less predictable, as greenhouse gas emissions from agriculture need to be cut, and as land and water resources are shrinking or deteriorating. Whilst technology will undoubtedly hold many of the keys to long-term global food security, there is a lot we can do today with existing knowledge (Fig. 2).
To make agriculture more productive and profitable in the face of rising costs and rising standards of human and environmental health, the best combination of available technologies has to be used. Much of the increases in yield per unit of area can be attributed to more efficient control of (biotic) stress rather than an increase in yield potential. The reduction of current yield losses caused by pests, pathogens and weeds are major challenges to agricultural production (Oerke and Dehne 2004). The intensity of crop protection has increased considerably as exemplified by a 15–20-fold increase in the amount of pesticides used worldwide (Oerke 2005).
Diverse ecosystems have been replaced in many regions by simple agro-ecosystems which are more vulnerable to pest attack. In order to safeguard the high level of food and feed productivity necessary to meet the increasing human demand, these crops require protection from pests (Popp 2011). Helping farmers lose less of their crops will be a key factor in promoting food security but even in the poorest countries whose rural farmers aspire to more than self-sufficiency. Food security is only the first step towards greater economic independence for farmers (FAO 2009).
The beneficial outcome from use of pesticides provides evidence that pesticides will continue to be a vital tool in the diverse range of technologies that can maintain and improve living standards for the people of the world. Some alternative methods may be more costly than conventional chemical-intensive agricultural practices, but often these comparisons fail to account for the high environmental and social costs of pesticide use. The externality problems associated with the human and environmental health effects of pesticides need to be addressed as well (National Research Council 2000).
Globally, agricultural producers apply around USD 40 billion worth of pesticides per annum. The market share of biopesticides is only 2 % of the global crop-protection market (McDougall 2010). Farmers in highly developed, industrialised countries expect a four- or fivefold return on money spent on pesticides (Gianessi and Reigner 2005; Gianessi and Reigner 2006; Gianessi 2009). Can we meet world food demands if producers continue, increase or discontinue pesticide use because of reduced economic benefits? Can better integrated pest management (IPM) preserve the economic benefits of pesticide use? These are just some of the questions facing scientists and pest management experts at a time when agriculture faces some of its greatest challenge in history between now and the year 2050 (Popp 2011).
Crop losses to pests
Crop productivity may be increased in many regions by high-yielding varieties, improved water and soil management, fertilisation and other cultivation techniques. An increased yield potential of crops, however, is often associated with higher vulnerability to pest attack leading to increasing absolute losses and loss rates (Oerke et al. 1994). An average of 35 % of potential crop yield is lost to pre-harvest pests worldwide (Oerke 2005).
In addition to the pre-harvest losses transport, pre-processing, storage, processing, packaging, marketing and plate waste losses along the whole food chain account for another 35 % (Fig. 3). In addition to reduce crop losses due to pests, avoiding waste along the whole length of the food chain is also a key (Popp 2011).
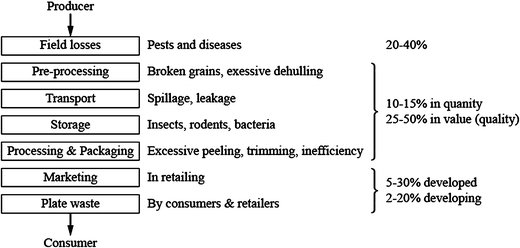
Losses along the food chain. An average of 35 % of potential crop yield is lost to pre-harvest pests worldwide. In addition to the pre-harvest losses transport, pre-processing, storage, processing, packaging, marketing and plate waste losses along the whole food chain account for another 35 %. Source: IWMI (2007)
Evolutionary interactions between pests and farmers predate conventional pesticides by thousands of years. Various loss levels may be differentiated, e.g. direct and indirect losses or primary and secondary losses, indicating that pests not only endanger crop productivity and reduce the farmer's net income but may also affect the supply of food and feed as well as the economies of rural areas and even countries (Zadoks and Schein 1979). Weeds affect crop productivity especially due to the competition for inorganic nutrients (Boote et al. 1983). Crop protection has been developed for the prevention and control of crop losses due to pests in the field (pre-harvest losses) and during storage (post-harvest losses). This paper concentrates on pre-harvest losses, i.e. the effect of pests on crop production in the field and the effect of control measures applied by farmers in order to minimise losses to an acceptable level (Oerke 2005).
An assessment of the full range of agricultural pests and of the composition and deployment of chemical pesticides to control pests in various environments would be an impossible task because of the large volume of data and the number of analyses required to generate a credible evaluation. The assessment of crop losses is important for demonstrating where future action is needed and for decision making by farmers as well as at the governmental level. According to German authorities in 1929, animal pests and fungal pathogens each caused a 10 % loss of cereal yield. In potato, pathogens and animal pests reduced production by 25 % and 5 %, respectively; while in sugar beet, production was reduced by 5 % and 10 % due to pathogens and animal pests, respectively (Morstatt 1929). In the USA, in the early 1900s, pre-harvest losses caused by insect pests were estimated at seldom less than 10 % (Marlatt 1904). Later, the United States Department of Agriculture published data on pre-harvest losses in 1927, 1931, 1939, 1954 and 1965 (Cramer 1967). However, the loss data became outdated due to significant changes in area harvested, production systems and intensity, control options and product prices.
Estimates of actual losses in crop production worldwide were updated nearly 30 years later for the period 1988–90 on a regional basis for 17 regions by Oerke et al. (1994). Increased agricultural pesticide use nearly doubled food crop harvests from 42 % of the theoretical worldwide yield in 1965 to 70 % of the theoretical yield by 1990. Unfortunately, 30 % of the theoretical yield was still being lost because the use of effective pest-management methods was not applied uniformly around the world and it still is not. Without pesticides, 70 % of crop yields could have been lost to pests (Oerke 2005).
Since crop production technology and especially crop-protection methods are changing continuously, loss data for eight major food and cash crops—wheat, rice, maize, barley, potatoes, soybeans, sugar beet and cotton—have been updated for the period 1996–98 on a regional basis for 17 regions (Oerke and Dehne 2004). Among crops, the loss potential of pests worldwide varied from less than 50 % (on barley) to more than 80 % (on sugar beet and cotton). Actual losses were estimated at 26–30 % for sugar beet, barley, soybean, wheat and cotton, and 35 %, 39 % and 40 % for maize, potatoes and rice, respectively (Oerke and Dehne 2004).
Since the early 1990s, production systems and especially crop-protection methods have changed significantly, especially in crops like maize, soybean and cotton, in which the advent of transgenic varieties has modified the strategies for pest control in some major production regions. Loss data for major food and cash crops have been updated most recently by Commonwealth Agricultural Bureaux International's Crop Protection Compendium for six food and cash crops—wheat, rice, maize, potatoes, soybeans and cotton—for the period 2001–2003 on a regional basis (CABI's Crop Protection Compendium 2005; Oerke 2005). Nineteen regions were specified according to the intensity of crop production and the production conditions. Among crops, the total global potential loss due to pests varied from about 50 % in wheat to more than 80 % in cotton production. The responses are estimated as losses of 26–29 % for soybean, wheat and cotton, and 31 %, 37 % and 40 % for maize, rice and potatoes, respectively.
Worldwide estimates for losses to pests in 1996–98 and 2001–03 differ significantly from estimates published earlier (Cramer 1967; Oerke et al. 1994). Obsolete information from old reports has been replaced by new data. Alterations in the share of regions differing in loss rates in total production worldwide are also responsible for differences (Table 1). Moreover, the intensity and efficacy of crop protection has increased since the late 1980s especially in Asia and Latin America where the use of pesticides increased above the global average (Yudelman et al. 1998).
Estimates of pesticide-related productivity
The increased threat of higher crop losses to pests has to be counteracted by improved crop protection whatever method it will be (biologically, mechanically, chemically, IPM and training of farmers). The use of pesticides has increased dramatically since the early 1960s; in the same period also, the yield average of wheat, rice and maize, the major sources for human nutrition, has more than doubled. Without pesticides, food production would drop and food prices would soar. With lower production and higher prices, farmers would be less competitive in global markets for major commodities.
Where overall crop productivity is low, crop protection is largely limited to some weed control, and actual losses to pests may account for more than 50 % of the attainable production (Oerke 2005). In large parts of Asia and Latin America, great advances have been made in the education of farmers, whereas the situation is still poor in Sub-Saharan Africa and has worsened in the countries of the former Soviet Union because of the lack of resources. (McDougall 2010).
Use patterns of pesticides vary with crop type, locality, climate and user needs. Plant disease can be devastating for crop production, as was tragically illustrated in the Irish potato famine of 1845–1847. This disaster led to the development of the science of plant pathology (Agrios 1988). From the time when synthetic pesticides were developed after World War II, there have been major increases in agricultural productivity accompanied by an increase in efficiency, with fewer farmers on fewer farms producing more food for more people. A major factor in the changing productivity patterns, either directly or indirectly, has been the use of pesticides.
Ensuring the safety and quality of foods and the increase in crop loss was accompanied by a growth in the rate of pesticides use. The annual global chemical-pesticide market is about 3 million tonnes associated with expenditures around USD 40 billion (Popp 2011). The growing dependence on chemical pesticides has been called the "pesticide treadmill" by entomologists (Bosch 1978). A major factor in the "pesticide treadmill" involves two responses to pesticide resistance. The first is to increase the dose and frequency of use of the less effective pesticide; this typically results in higher levels of pest resistance and damage to natural enemies and the environment. The second response is to develop and commercialise a new pesticide. The treadmill concept assumes that this two-step process will continue until the pest meets a resistance-proof pesticide or until the supply of effective new pesticides is exhausted. The greater the impact of control measures on pest populations, the more extreme are their evolutionary responses. However, the moderate rates in yield increase in the major world crops during 1965–2000 did not offer a strong case for a high increase in pesticide use even taking into account the fair amount of change in the cropping systems of developing countries with an expansion of the fruits and vegetable sector (FAO 2000).
Pesticide productivity has been estimated in three general ways: with partial-budget models based on agronomic projections, with combinations of budget and market models, and with econometric models. For a long time, benefit analyses relied on partial budgeting. The most widely cited studies on pesticide productivity, those of Pimentel and various coauthors, use this method (Pimentel et al. 1978, 1991, 1992). Assessment of global crop losses by Cramer (1967) also falls into this category, as does with Knutson et al. (1993). Those studies use data from field trials and expert opinion to estimate pest-induced losses on crop by crop basis with current pesticide use, without pesticides, and with a 50 % reduction in pesticide use. They construct alternative production scenarios for each crop to estimate changes in input use. Current prices are then used to value changes in per-acre production costs and per-acre yield losses, which are added to obtain an estimate of the costs of changes in pesticide use. One of these studies (Pimentel et al. 1991) estimates that aggregate crop losses amounted to 37 % of total output in 1986, up from 33 % in 1974. In comparison, Cramer (1967) estimated crop losses of around 28 % due to all pests in all of North and Central America. Estimates of crop losses at 37 % are questionably high. The costs of pesticides are low relative to crop prices and total production costs. Crop losses of the magnitude estimated by Pimentel et al. (1991) should be sufficient to make it profitable to use chemical pest controls at much greater rates than observed today.
Partial-budget models of this kind generally overstate pesticide productivity and thus the economic effects of changes in pesticide use because they consider only a small subset of substitution possibilities (Lichtenberg et al. 1988). The models ignore even short-run, farm-level substitution possibilities caused by differences in land quality, human capital, and other characteristics of farm operations. Field trials can hold constant all production practices except pesticide use, deliberately ignoring substitution possibilities. Moreover, they are often conducted in areas with heavier than normal pest pressure, where pesticide productivity is probably higher (Pimentel et al. 1991). As a result, studies on crop losses due to pests based on partial-budget models tend to overestimate crop losses in agriculture.
Other studies have attempted to estimate pesticide-related effects of large reductions in pesticide use by combining partial-budget models with models of output markets (Zilberman et al. 1991; Ball et al. 1997). These studies use the same approach as partial-budget models in estimating yield and cost effects of changes in pesticide use. Projected changes in per-hectare expenses and yields are then incorporated into models of agricultural-commodity markets and used to project changes in output prices and consumption in market equilibrium. Models of this type incorporate some, but by no means all, substitution possibilities. The productivity of pesticides—and thus the effects of reducing pesticide use—depends in large measure on substitution possibilities within the agricultural economy (Zilberman et al. 1991). In general, pesticide productivity will tend to be low in situations where substitution possibilities are large. Real prices of energy and durable equipment have fallen relative to agricultural chemical prices (Ball et al. 1997). On the other hand, the prices of hired and self-employed labour have risen steadily, both in real terms and relative to agricultural chemical prices, and this suggests that labour-intensive pest-control methods have become less attractive relative to pesticide use. However, those estimates failed to take into account the possibility that other pest-control strategies could be used or that new technologies could be developed in the absence of chemical control. Moreover, pesticide use can improve food quality in storage and provides some benefits directly to consumers. Zilberman et al. (1991) estimated that every dollar increase in pesticide expenditure raises gross agricultural output by USD 3–6. Most of that benefit is passed on to consumers in the form of lower prices for food.
It is possible to estimate pesticide productivity directly with econometric models. Statistical methods can be used to estimate parameters of models that link output with input use. Varied substitution possibilities are implicit in the parameters of these models. Specification of models that are nonlinear in input use allows rates of substitution between inputs to vary as input usage changes. Econometric models are commonly used to estimate factor productivity and productivity growth in the agricultural economy (Griliches 1963; Ball 1985; Capalbo and Antle 1988; Chavas and Cox 1988; Fernandez-Cornejo et al. 1998; Chambers and Pope 1994). Econometric models capture all forms of substitution in production, including short-term and long-term substitutes for pesticides on individual farms and at the regional and national levels.
Headley (1968) estimated such a model by using state-level cross-sectional data in the US for the year 1963. He used crop sales to measure output and expenditures on fertilisers, labour, land and buildings, machinery, pesticides and other inputs as measures of input use and found that an additional dollar spent on pesticides increased the value of output by about USD 4 showing a high level of productivity for that period. There are several reasons to believe that Headley's estimate of marginal pesticide productivity could be too high. Firstly, using sales as a measure of output tends to bias productivity estimates upward because output price tends to be positively correlated with input demand. Secondly, Headley's specification assumes that pesticides are an essential input, that is, that production is impossible without pesticides. Finally, the specification that Headley uses does not allow pesticide productivity to decline as fast as it should, again leading to upwardly biased estimates of pesticide productivity (Lichtenberg and Zilberman 1986). The Headley model generates estimates of the marginal productivity associated with pesticides, that is, the additional amount (value) of output obtained by using an additional unit of pesticides. Multiplying the marginal productivity of pesticides by the quantity of pesticides used thus understates the total value added by pesticides (Pimentel et al. 1992).
Carrasco-Tauber and Moffitt (1992) applied this approach to state-level cross-sectional data on sales and input expenditures in the U.S. like those used by Headley (1968). Their use of sales as a dependent variable generated an implicit estimate of aggregate US crop losses in 1987 of 7.3 % at average pesticide use, far less than estimates of other studies (Pimentel et al. 1991; Oerke et al. 1994). That specification suggests that their estimate of pesticide productivity should be biased upward. Chambers and Lichtenberg (1994) developed a dual form of this model based on the assumptions of profit maximisation and separability between normal and damage-control inputs. They used this dual formulation to specify production relationships under two specifications of damage abatement, neither of which imposed the assumption that pesticides are essential inputs. Implicit crop losses in 1987 estimated from those models ranged from 9 % to 11 %, only about one quarter to one third of the size estimated by others (Pimentel et al. 1991; Oerke et al. 1994). Assuming no change in crop prices, farm income would decrease by 6 %, considerably more than estimated by other studies (Pimentel et al. 1991; Oerke et al. 1994). Estimated crop losses with zero pesticide use ranged from 17 % to 20 %.
Costs and benefits of pesticide use
The economic analyses of pesticide benefits is hindered by the lack of pesticide use data and economic models for minor crops and non-agricultural pesticides. Cost–benefit analysis is increasingly used to assess resource management and environmental policies. This approach monetises all costs and benefits so that they are measured in currencies and its full implementation might be constrained by data limitations and difficulties in monetising human and environmental health risks. Economic impacts are further complicated by the various governmental programmes that subsidise pesticide users, such as price supports and deficiency payments.
The most commonly recognised economic incentives are based on the "polluter pays" principle, including the use of licensing fees, user fees or taxes. The experience of those countries (Denmark, Sweden and Norway) that have introduced these taxes is that they appear to have played some role in reducing pesticide use. However, their price elasticity estimates are low and this suggests comparatively little effect in terms of quantity reductions, unless they are set at very high rates relative to price. There is some suggestion that revenue recycling may have been more effective, with revenues redirected to research and information. Using revenues to further research or encourage changes in farming practice would appear to make more sense (Pearce and Koundouri 2003).
Pesticides vary in their toxicity by design and also according to the conditions in the receiving environment. The theoretical solution here is to express the tax as an absolute sum per unit of toxicity-weighted ingredient. Unfortunately, there are few examples (the Norwegian reforms of 1999) of actual taxes being differentiated by toxicity. Even though the overall demand for pesticides is not reduced significantly by a tax, a toxicity-differentiated tax may be effective if substitution between pesticides will occur in such a way that the overall toxic impact of pesticides will be reduced. It means that pesticide use and toxicity could be "decoupled" by a pesticide tax. The problem with pesticide tax studies is that few of them simulate the "cross-price effects" of such a policy, i.e. they do not look closely at substitution between types of pesticides (or between pesticides and other inputs such as fertilisers and land). Simulations of such toxicity-weighted taxes for the UK show that overall price elasticity of demand for pesticides was consistently low and never greater than −0.39. However, cross-price elasticities between the "banded" pesticides (banded according to toxicity) were greater than the "own" price elasticities, suggesting that farmers might switch between types of pesticide (Pearce and Koundouri 2003).
Nevertheless, the "polluter pays" principle (i.e. adding the environmental and public health costs to the price paid by consumers) can be an effective approach to internalise the social costs of pesticide use. The fees and taxes generated can be used to promote improved (sustainable) pest management. In order to set the right level of levies and taxes, it may be necessary to calculate the negative impacts of pesticides. Various attempts have been made to determine the costs that relate to public health (risks to farm workers and consumers and drift risk) and damage to beneficial species, and to the environment (Pimentel et al. 1992; Pimentel and Greiner 1997; Pimentel 2005).
However, pesticides can result in a range of benefits including wider social outcomes with benefits being manifested in increased income and reduced risk, plus the ability to hire labour and provide employment opportunities. Other outcomes were the evolution of more complex community facilities, such as schools and shops and improved health (Bennett at al. 2010).
The costs of pesticides and non-chemical pest-control methods alike are low relative to crop prices and total production costs. Pesticides account for about 7–8 % of total farm production costs in the EU. However, there is wide variation among Member States fluctuating between 11 % in France and Ireland and 4 % in Slovenia (Popp 2011). Pesticides account for 5–6 % of total farm input in monetary terms in the USA (USDA 2010).
Overall, farmers have sound economic reasons for using pesticides on crop land. The global chemical-pesticide market is about 3 million tonnes associated with expenditures around USD 40 billion in a year. As a result of the increasing use of GM herbicide-tolerant and insect-resistant crop seed and sales of agrochemicals used in non-crop situations (gardening, household use, golf courses, etc), the value of the overall crop-protection sector is estimated to reach about USD 55 billion. The increasing sale of GM seeds has had a direct impact on the market for conventional agrochemical products (McDougall 2010). In spite of the yearly investments of nearly USD 40 billion worldwide, pests cause an estimated 35 % actual loss (Oerke 2005). The value of this crop loss is estimated to be USD 2000 billion per year, yet there is still about USD 5 return per dollar invested in pesticide control (Pimentel 2009).
According to the national pesticide benefit studies in the United States, USD 9.2 billion are spent on pesticides and their application for crop use every year (Gianessi and Reigner 2005; Gianessi and Reigner 2006; Gianessi 2009). This pesticide use saves around USD 60 billion on crops that otherwise would be lost to pest destruction. It indicates a net return of USD 6.5 for every dollar that growers spent on pesticides and their application. However, the USD 60 billion saved does not take into account the external costs associated with the application of pesticides in crops (Table 2).
The correct use of pesticides can deliver significant socio-economic and environmental benefits in the form of safe, healthy, affordable food; contribute to secure farm incomes and enable sustainable farm management by improving the efficiency with which we use natural resources such as soil, water and overall land use. Obviously, when pesticides are not used correctly, then the socio-economic and environmental benefits may not be realised and the economic damage resulting from widespread pesticide use should also be highlighted. The environmental and public health costs of pesticides necessitate the consideration of other trade-offs involving environmental quality and public health when assessing the net returns of pesticide usage. Pimentel et al. (1992) found that pesticides indirectly cost the U.S. USD 8.1 billion a year including losses from increased pest resistance; loss of natural pollinators (including bees and butterflies) and pest predators; crop, fish and bird losses; groundwater contamination; and harm to pets, livestock and public health. In a supplementary study, Pimentel (2005) estimates that the total indirect costs of pesticide use was around USD 9.6 billion in 2005. Had the full environmental, public health and social costs been included the total cost could have risen to USD 9.6 billion figure (Pimentel 2005). It means that past assessments of environmental and social impact have been narrow and should they be broadened to USD 20 billion per year the previous estimate of USD 60 billion worth of production benefits to the U.S. from pesticide use would be dramatically lower (USD 40 billion) if net effects are considered. However, the net benefit still shows a high profitability of pesticides indicating a net return of USD 3 for every dollar spent on pesticides (Popp 2011).
Genetically engineered organisms that reduce pest pressure constitute a "new generation" of pest-management tools. Biotechnology has delivered economic and environmental gains through a combination of their inherent technical advances and the role of the technology in the facilitation and evolution of more cost-effective and environmentally friendly farming practices. This change in production system has made additional positive economic contributions to farmers and delivered important environmental benefits, notably reduced levels of GHG emissions. The Environmental Impact Quotient (EIQ) distils the various environmental and health impacts of individual pesticides in different GM and conventional production systems into a single "field value per hectare" and draws on key toxicity and environmental exposure data related to individual products. The environmental impact associated with herbicide and insecticide use on GM crops, as measured by the EIQ indicator fell by 16.3 %. During the period 1996 to 2008, pesticide reduction was estimated at 356 million kilogram of active ingredient, a saving of 8.4 % in pesticides (Brookes and Barfoot 2010).
Pesticides effectively control many insects, diseases and weeds. However, to be effective, pesticides have to target the crop or animal of interest. Spray drift is one of the biggest concerns regarding the movement of pesticides to non-target organisms. Off-target losses can range from 50 % to 70 % of the applied pesticide because of evaporation and drift (Pimentel 2005). Drift from aerial applications is greatest and that from ground applications is least. There are several ways to reduce drift. One way is to use spray additives that affect the drop size of sprays by increasing the number of large droplets and decreasing the number of small droplets (Hall and Fox 1997). Another method to decrease the number of fine droplets during spraying is to use new nozzles that are designed to decrease the number of fine droplets. The nozzles work by increasing droplet size through a reduction in the velocity of the liquid just before it is discharged (Ozkan 1997).
Biopecticides and integrated pest management
Biological control is urgently needed, opening increasing possibilities for biopesticides. Biopesticides offer important social benefits, as compared with conventional pesticides. Yet in an agricultural industry that is still dominated by pesticides, biological control has found its place in the form of augmentative releases, particularly for the management of pests that are difficult to control with insecticides. Since pest problems in agriculture involve plants, plant-feeding organisms and their natural enemies, the regulation of biological control agents has usually been the responsibility of national plant quarantine services. For this reason, regulation over several decades focused on the need to ensure that introduced natural enemies would not become agricultural pests (Waage 1997).
There has been a strong tendency to consider biopesticides as "chemical clones" rather than as biological control agents, and therefore the chemical pesticide model has been followed. On the other hand, regulation of biopesticides is needed because being "natural" does not mean it is safe. However, the challenge of new and more stringent chemical pesticide regulations, combined with increasing demand for agriculture products with positive environmental and safety profiles, is boosting interest in biopesticides. It takes an average of 3 to 6 years and USD 15–20 million to develop and register a biopesticide compared with 10 years and USD 200 million for synthetic pesticides (REBECA 2007). Many of the major pesticide manufacturers are jumping into the biopesticide industry. This wider recognition of biopesticides is partly in response to major food buyers like Sysco, Wal-Mart and McDonald's requesting suppliers use "sustainable" agricultural practices.
Global sales of biopesticides are estimated to total around USD 1 billion, still small compared to the USD 40 billion in the worldwide pesticide market. It is pegged at around 2 % of the global crop-protection market (Popp 2011). While biopesticides may be safer than conventional pesticides, the industry is composed mostly of small- to medium-sized enterprises, and it is difficult for one company to fully and comprehensively fund research and development, field development and provide the marketing services required to make a successful biopesticide company. Another challenge is the lack of innovative biopesticide products coming to the marketplace and their registration (Farm Chemical Internationals 2010).
Large agrochemical companies are getting more and more involved in ecologically based IPM. For example, the stewardship team of Syngenta turned a thought leadership idea into a project: MARGINS—Managing Agricultural Runoff into Surface Water. Field margins are not only essential to help reducing some of the risks associated with the use of pesticides but can play several important roles. They can be windbreaks to protect crops and soil; can influence the flow of nutrients and water within the landscape; provide controlled access in the countryside whilst leaving the cultivated area undisturbed; or can enhance the visual appearance of the countryside with flower strips feeding of pollen and nectar the pollinating insects. Furthermore, field margins can also be specifically managed to enhance game bird populations, by providing nesting cover and food resources, and provide over-wintering habitat, or refuges, for many insects—in some instances beneficial predators. The main aim of the MARGINS project is to demonstrate the integration of crop productivity needs with the demands for protecting water, biodiversity and soil since crop production depends on finite soil resources being kept in good condition. As a start-up pilot, the project was initiated in 2009 near Lake Balaton in Hungary (Szentgyörgyvár)—the largest lake in Central Europe—which is renowned for its beauty and wildlife, but which is surrounded by steep rolling hills of very productive loam soils that are prone to accelerated runoff. Conservation tillage resulted in the lowest pesticide levels in runoff; it doubled when there was a bare buffer strip at the bottom of the plot (Fig. 4). The buffer strips are well established with a thick sward of clover and other flowering plants (Syngenta 2010).
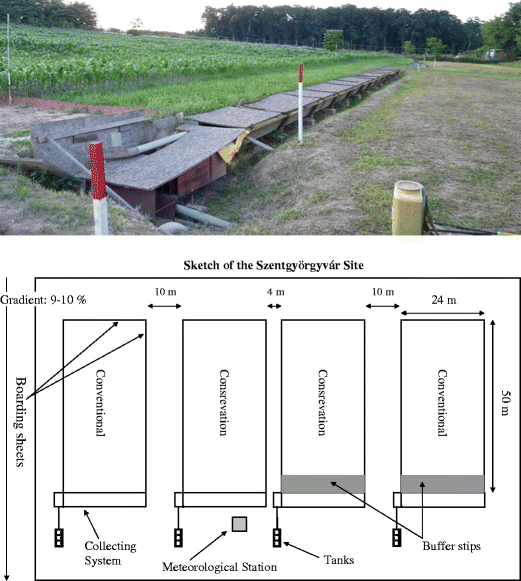
MARGINS—Managing Agricultural Runoff into Surface Water. Margins is in-field and edge-of-field management with four combinations (conservation till vs conventional till plus vegetative buffers) to enable ranking of relative effectiveness. Source: Syngenta (2010)
These results are also consistent with the previous project, SOWAP (Soil and Water Protection), conducted on these field plots. This project (supported by EU Life+) demonstrated that conservation tillage consistently reduced runoff, soil erosion and soil nutrient losses. In addition, numbers of earthworms, beetles and other soil fauna increased, as did microbial biomass activity. But there were also benefits for farmers because profitability was maintained. Crop establishment costs were reduced by 15–20 % in conservation tillage. However, crop yields were slightly lower, as commonly found during the conversion to conservation tillage. Nevertheless, they were higher in dry years, since water availability increased due to reduced runoff from conservation tillage (Syngenta 2010).
This start-up pilot is encouraging. Syngenta's hope is to extend this project across Europe to other landscapes and land use patterns, particularly where it shows how to implement CAP reform via agri-environment incentives. The next paradigm shift in agriculture needs to be driven by continually looking for ways to work more productively with nature. MARGINS is an example of how to meet the demands of sustainable agriculture—a skillful blend of modern technology with respect for nature. Further research and development, along with investment in new technologies, is vital to maintain a sustainable, competitive agricultural industry which can still deliver the required economic, social and environmental benefits. Supporting technological progress and enhancing investments in research through the agricultural policy, along with the education to put developments into practice, will help a sustainable, competitive farming sector to balance productivity with the efficient use of natural resources and deliver economic and environmental public goods (Syngenta 2010).
During the past two decades, IPM programmes have reduced pest control costs and pesticide applications in fruit, vegetable and field crops. Reductions in pest control costs and pesticide use in IPM programmes can be achieved by introducing or increasing populations of natural enemies, variety selection, cultural controls, applying alternative pesticides and improving timing of pest suppression treatments. For farmers, very often the main benefit of IPM is the avoidance of uneconomical pesticide use. However, a large part of the benefits are reduction of externalities and therefore occur to other groups. This poses considerable measurement and valuation problems. Although the IPM programmes did reduce pesticide use, most of the programmes still relied heavily on pesticides.
However, new scientific knowledge and modern technologies provide considerable opportunities, even for developing countries, to further reduce current yield losses and minimise the future effects of climate change on plant health. Finding continuously new cost-effective and environmentally sound solutions to improve control of pest and disease problems is critical to improving the health and livelihoods of the poor. The need for a more holistic and modernised IPM approach in low-income countries is now more important than ever before. The institutional environment for IPM at the global level has become more complex. The trend towards market liberalisation in the absence of specific policy frameworks has not always been supportive to IPM. For the pesticide market, liberalisation without effective regulations and adequate market-based incentives may lower the costs of supplying pesticides, but at the same time can increase the tendency for ineffective, inefficient, and non-sustainable crop protection. For a system-wide programme on IPM to make a significant contribution, the policy and institutional environment of global crop protection cannot be ignored (Settle and Garba 2011). There is a danger that in the case of IPM the situation can be exploited by pesticide companies that use IPM as a marketing instrument to maximise sales of their chemical pesticides and biotechnology products.
However, the European Commission Directive 2009/128/EC on the sustainable use of pesticides establishes a framework to achieve a sustainable use of pesticides by reducing the risks and impacts of pesticide use on human health and the environment and promoting the use of IPM and alternative approaches or techniques such as non-chemical alternatives to pesticides. One of the key features of the Directive is that each Member State should develop and adopt its National Action Plan and set up quantitative objectives, targets, measures and timetables to reduce risks and impacts of pesticide use on human health and the environment and to encourage the development and introduction of integrated pest management and of alternative approaches or techniques in order to reduce dependency on the use of pesticides. Other provisions include compulsory testing of application equipment, training and certification of all professional users, distributors and advisors; a ban (subject to derogations) on aerial spraying; special measures to protect the aquatic environment, public spaces and conservation areas; minimising the risks to human health and the environment through handling, storage and disposal (Official Journal of the European Union 2009).
Challenges of the global pesticide market
Globalisation is affecting pest management on and off the farm. Reduction in trade barriers increases competitive pressures and provides extra incentives for farmers to reduce costs and increase crop yields. Liberalisation of input markets, often labelled as successful market reform, can lead to inefficient pesticide use and high external costs (FAO 2009). Other forms of trade barriers create disincentives for adopting new technologies such as the reluctance of the EU to accept genetically modified organisms.
It is important to point out that it is not only the big multinationals that are important players in pesticide policy but also the many new companies in developing countries who produce generics. A trend in agrichemical industry is the movement of many chemical pesticides off patent. As these chemicals become generic pesticides, manufacturers lose their monopolies on them. Overall, generic companies make up about 30 % of total sales (McDougall 2010). Rising sales of generic pesticides, especially in countries in Africa and Latin America but also in some Asian countries, is often facilitated by weak regulatory control and the lack of an IPM oriented national policy framework countries (FAO 2009).
Around 30 % of pesticides marketed in developing countries with an estimated market value of USD 900 million annually do not meet internationally accepted quality standards. They are posing a serious threat to human health and the environment. Such pesticides often contribute to the accumulation of obsolete pesticide stocks in developing countries (FAO 2009). Possible causes of low quality of pesticides can include both poor production and formulation and the inadequate selection of chemicals. When the quality of labelling and packaging is also taken into account, the proportion of poor-quality pesticide products in developing countries is even higher. Falsely declared products continue to find their way to markets for years without quality control (FAO 2002).
The problem of poor-quality pesticides is particularly widespread in sub-Saharan Africa, where quality control is generally weak. The UN agencies urged governments and international and regional organisations to adopt the worldwide accepted FAO/WHO pesticide specifications to ensure the production and trade of good quality products. Countries should make these voluntary standards legally binding. The FAO/WHO standards are especially important for developing countries that lack the infrastructure for proper evaluation of pesticide products. Pesticide industries, including producers of generic pesticides, should submit their products for quality assessment to FAO/WHO (FAO/WHO 2010). Another negative economic consequence of a higher use of pesticides in developing countries is the loss of export opportunities for developing countries especially with horticultural crops as the developed countries are tightening maximum residue levels. In turn, agricultural lobbyists in industrialised nations may exploit this situation and use environmental standards as non-tariff trade barriers.
Sustainable, IPM based on biological control is urgently needed, opening increasing possibilities for biopesticides. Their beneficial features include that they are often very specific, they are "inherently less toxic than conventional pesticides" compatible with other control agents, leave little or no residue, are relatively inexpensive to develop and support the action of natural enemies in ecologically based IPM. The market share of biopesticides is growing faster than that of conventional chemicals. In recent times, large agricultural chemical companies have become very dynamic and are constantly on the lookout for technology that complements what they already have or that complements a segment of the market that they are focused on. While biopesticides are typically seen as an alternative to synthetic chemicals, some experts see biopesticides as complementary to conventional pesticides already on the market. Increasing demand for chemical-free crops and more organic farming has led to augmented usage of biopesticides in North America and Western Europe (ICIS 2009). Key factors in this growth include a larger overall investment in biopesticide research and development, a more established application of IPM concept and an increased area under organic production. Products not requiring registration and products which already have been registered have priority in the research and development of these companies.
As a result of the various merger and acquisition that have taken place, the agrochemical sector is relatively highly consolidated. An increasing number of merger and acquisition transactions have been targeted at strengthening the respective product portfolios of the purchasing company through the acquisition of a particular agrochemical product or product range. While product acquisitions have always been a feature of the agrochemical industry, the overall level of this type of merger and acquisition activity has increased significantly in the last 10 years (McDougall 2010).
The total cost of agrochemical research and development expenditure in 2007 for 14 leading companies was 6.7 % of their agrochemical sales. Over the next 5 years, it is expected that herbicides will lead market growth while the insecticides sector is likely to suffer further generic pressure and the fungicide sector is expected to grow relatively modestly with increases generated from a further expansion of the seed treatment sector. The GM crop sector is also expected to continue to move increasingly toward multiple trait stacked gene varieties, in both established and developing markets (McDougall 2010).
Industrial leaders expect that advances in genomics will lead researchers to the precise location and sequence of genes that contain valuable input and output traits. A shift in research and development resources from input to output traits probably would have a large impact on the future of plant protection. Will the cycle of innovation on the input side continue? Because of the high investment required for development of chemical pesticides and transgenic crops, will large agrichemical and life-science firms focus primarily on crops with large markets? Whether companies will develop pesticides and input traits for minor use crops remains an open question. These are the main questions research and development of plant protection is facing at present.
Conclusions
The main reasons why world food supply is tightening are population growth, accelerated urbanisation and motorisation, changes in diets and climate change. Furthermore, agricultural land is used to produce more bioenergy and other bio-based commodities. To meet the increasing world food demand, the necessary production growth will to a large extent have to be met by a rise in the productivity of the land already being farmed today. However, this will be difficult to accomplish as global agricultural productivity growth has been in decline since the Green Revolution. In addition to the reduction of waste along the whole food chain priority has to be given to effective crop-protection measures to cut further crop losses to pests.
Cost–benefit analyses are important tools for informing policy decisions regarding use of chemical pesticides. The impacts of pesticides on the economy, environment, and public health are measured in monetary terms. However, there are many uncertainties in measuring the full array of benefits and costs of pesticide use. Making wise tradeoffs to achieve a fair balance between the risks that a community bears and the benefits that it receives is one of the most difficult challenges for policy makers.
Chemical pesticides will continue to play a role in pest management because environmental compatibility of products is increasing and competitive alternatives are not universally available. Pesticides provide economic benefits to producers and by extension to consumers. One of the major benefits of pesticides is protection of crop quality and yield. Pesticides can prevent large crop losses, thus raising agricultural output and farm income. The benefits of pesticide use are high-relative to risks. Non-target effects of exposure of humans and the environment to pesticide residues are a continuing concern. Side effects of pesticides can be reduced by improving application technologies. Innovations in pesticide-delivery systems in plants promise to reduce adverse environmental impacts even further but are not expected to eliminate them. The correct use of pesticides can deliver significant socio-economic and environmental benefits.
The justifications of government intervention in the management of pest control include the need to address the externality problems associated with the human and environmental health effects of pesticides. However, few incentives exist for efficient and environmentally sound pest control management strategies. Such incentives as taxes and fees for the use of various categories of chemicals have been recommended in some countries but the overall demand for pesticides is not reduced significantly. However, in the area of plant protection products, further measures regarding information on and safe handling of pesticides have been laid down recently in a framework for Community action to achieve the sustainable use of pesticides was established by the Directive 2009/128/EC.
Genetically engineered organisms that reduce pest pressure constitute a "new generation" of pest-management tools. This change in production system has made additional positive economic contributions to farmers and delivered important environmental benefits. But genetically engineered crops that express a control chemical can exert strong selection for resistance in pests. Thus, the use of transgenic crops will even increase the need for effective resistance-management programmes.
Many biocontrol agents are not considered acceptable by farmers because they are evaluated for their immediate impact on pests. Evaluation of the effectiveness of biocontrol agents should involve consideration of long-term impacts rather than only short-term yield, as is typically done for conventional practices. The global sale of biopesticides is very small compared to the pesticide market. However, the market share of biopesticides is growing faster than that of conventional chemicals. A concerted effort in research and policy should be made to increase the competitiveness of alternatives to chemical pesticides for diversifying the pest-management "toolbox". But availability of alternative pest-management tools will be critical to meet the production standards and stiff competition is expected in these niche markets.
New scientific knowledge and modern technologies provide considerable opportunities, even for developing countries, to further reduce current yield losses and minimise the future effects of climate change on plant health. Finding continuously new cost-effective and environmentally sound solutions to improve control of pest and disease problems is critical to improving the health and livelihoods of the poor. The need for a more holistic and modernised IPM approach in low-income countries is now more important than ever before.
Total investment in pest management and the rate of new discoveries should be increased to address biological, biochemical and chemical research that can be applied to ecologically based pest management. There is underinvestment from a social perspective in private-sector research because companies will aim to maximise only what is called suppliers' surplus. Companies will compare their expected profits from their patented products resulting from research and will not consider the benefits to consumers and users. Investments in research by the public sector should emphasise those areas of pest management that are not being undertaken by private industry. Transmission of knowledge in the past was the responsibility mostly of the public sector, but it has become more privatised. The public sector must act on its responsibility to provide quality education to ensure well-informed decision making in both the private and public sectors by emphasising systems-based interdisciplinary research.
References
-
Agrios GN (1988) Plant Pathology, 3rd edn. Academic Press, San Diego, 803 p
-
Ball VE (1985) Output, input and productivity measurement in US agriculture. Am J Ag Ec 67:476–486
-
Ball VE et al (1997) Agricultural productivity revisited. Am J Agric Econ 79:1045–1063
-
Bennett B et al (2010) We know where the shoe pinches: a case study-based analysis of the social benefits of pesticides. Outlook on Agriculture 39(9):79–87, IP Publishing Ltd
-
Boote KJ et al (1983) Coupling pests to crop growth simulators to predict yield reductions. Phytopathology 73:1581–1587
-
Brookes G, Barfoot P (2010) GM crops: global socio-economic and environmental impacts 1996–2008. PG Economics Ltd, UK
-
CABI's Crop Protection Compendium (2005) Commonwealth Agricultural Bureaux International (CABI). www.cabi.org/cpc (accessed 29 October 2011).
-
Capalbo SM, Antle JM (eds) (1988) Agricultural productivity: measurement and explanation. Resources for the Future, Washington, DC
-
Carrasso-Tauber C, Moffit LJ (1992) Damage control econometrics: functional specification and pesticide productivity. Am J Ag Ec 74:158–162
-
Chambers RG, Lichtenberg E (1994) Pesticide productivity and pest damage in the United States: an aggregate analysis. In: Willis CE, Zilberman D (eds) The economics of pesticides and food safety: essays in memory of Carolyn Harper. Kluwer Academic Publishing, Boston
-
Chambers RG, Pope RD (1994) A virtually ideal production system: specifying and estimating the vips model. Am J Ag Ec 76:105–113
-
Chavas JP, Cox T (1988) A nonparametric analysis of agricultural technology. Am J Ag Ec 70:303–310
-
Cramer HH (1967) Plant protection and world crop production. Bayer Pflanzenschutz-Nachrichten, 20: 1-524.European Commission (2010) Farm Accountancy Data Network, FADN Public Database. http://ec.europa.eu/agriculture/rica/database (accessed 29 September 2011)
-
FAO (2000) Report of the expert consultation on viticulture (Grape production) in Asia and the Pacific. Food and agriculture organization of the United Nations. FAO Regional Office for Asia and the Pacific, Bangkok
-
FAO (2002) The international code of conduct on the distribution and use of pesticides. Food and Agriculture Organization of the United Nations, Rome, 2002
-
FAO (2009) Feeding the world in 2050. World agricultural summit on food security 16–18 November 2009. Food and Agriculture Organization of the United Nations, Rome
-
FAO/WHO (2010) Manual on the development and use of FAO and WHO specifications for pesticides. Prepared by the FAO/WHO joint meeting on pesticide specifications (JMPS). World Health Organization—Food and Agriculture Organization of the United Nations, Rome
-
Farm Chemical Internationals (2010) Biological pesticides on the rise. www.farmchemicalsinternational.com/magazine (accessed 25 September 2010)
-
Fernandez-Cornejo J et al (1998) Issues in the economics of pesticide use in agriculture: a review of the empirical evidence. Rev Agric Econ 20(2):462–488
-
Gianessi LP (2009) The value of insecticides in U.S. crop production. Croplife Foundation, Crop Protection Research Institute (CPRI), March 2009
-
Gianessi LP, Reigner N (2005) The value of fungicides in U.S. crop production. Croplife Foundation, Crop Protection Research Institute (CPRI), September 2005
-
Gianessi LP, Reigner N (2006) The value of herbicides in U.S. crop production. 2005 Update. Croplife Foundation, Crop Protection Research Institute (CPRI), June 2006
-
Griliches Z (1963) The sources of measured productivity growth: US agriculture, 1940–1960. J Pol Ec 71:331–364
-
Hall FR, Fox RD (1997) The reduction of pesticide drift. In: Foy CL, Pritchard DW (eds) Pesticide formulation and adjuvant technology. CRC Press, Boca Raton, pp 209–240
-
Headly JC (1968) Estimating the productivity of agricultural pesticides. Am J Ag Ec 50:13–23
-
ICIS CBA (2009), Agriculture chemical firms' interest in biopesticides rises. ICIS Chemical Business Association. www.icis.com/Articles/2009/06/16 (accessed 25 September 2010)
-
IWMI (2007) Water for food, water for life: a comprehensive assessment of water management in agriculture. Earthscan and Colombo. International Water Management Institute, London
-
Knutson RD, Hall CR, Smith EG, Cotner SD, Miller JW (1993) Economic impacts of reduced pesticide use on fruits and vegetables. American Farm Bureau Federation, Washington, DC
-
Lichtenberg E, Zilberman D (1986) The welfare economics of regulation in revenue-supported industries: the case of price supports in U.S. agriculture. Am Econ Rev 76(5):1135–1141
-
Lichtenberg E et al (1988) Marginal analysis of welfare effects of environmental policies: the case of pesticide regulation. Am J Agric Econ 70:867–874
-
Marlatt CL (1904) The annual loss occasioned by destructive insects in the United States. United States Department of Agriculture, Yearbook, 461–474
-
McDougall (2010) Phillips McDougall, AgriService, Industry Overview—2009 Market, Vineyard Business Centre Saughland Pathhead Midlothian EH37 5XP Copyright 2010
-
Morstatt H (1929) Die jährlichen ernteverluste durch pflanzenkrankheiten und schädlinge und ihre statistischeermittlung. Bericht über Landwirtsch 9:433–477
-
National Research Council (2000) The future role of pesticides in US agriculture. National Academy Press, Washington, D.C
-
Oerke EC (2005) Crop losses to pests. J Agr Sci 144:31–43. doi:10.1017/S0021859605005708
-
Oerke EC, Dehne HW (2004) Safeguarding production—losses in major crops and the role of crop protection. Crop Prot 23:275–285
-
Oerke EC, Dehne HW, Schonbeck F, Weber A (1994) Crop production and crop protection—estimated losses in major food and cash crops. Elsevier Science, Amsterdam, 808 pp
-
Official Journal of the European Union (2009) Directive 2009/128/EC of the European Parliament and of the Council of 21 October 2009 establishing a framework for Community action to achieve the sustainable use of pesticides. http://eur-lex.europa.eu/JOHtml.do?uri=OJ:L:2009:309:SOM:EN:HTML (accessed 26 November 2011)
-
Ozkan E ed (1997) Equipment Developments. Chemical Application Technology, Newsletter 1:6p. http://www.ag.ohio-state.edu/catnews/catequi.htm (accessed 20 October 2011)
-
Pearce D, Koundouri P (2003) Fertilizer and Pesticide Taxes for Controlling Non-point Agricultural Pollution. Agriculture and Rural Development. The World Bank Group. http://www.worldbank.org/rural (accessed 04 November 2011)
-
Pimentel D (2005) Environmental and economic costs of the application of pesticides primarily in the United States. Environ Dev Sus 7:229–252
-
Pimentel D (2009) Pesticides and pest controls. In: Peshin R, Dhawan AK. (eds) (2009). Integrated pest management: Innovation-development process, 1: 83–87. Springer Science + Business Media B. V. 2009
-
Pimentel D, Greiner A (1997) Environmental and socio-economic costs of pesticide use, chapter 4. In: Pimentel D (ed) Techniques for reducing pesticide use: economic and environmental benefits. John Wiley and Sons, New York
-
Pimentel D et al (1978) Benefits and costs of pesticide use in US food production. BioScience 28(772):778–784
-
Pimentel D et al (1991) Environmental and economic impacts of reducing U.S. agricultural pesticide use. In: Pimentel D, Hanson AA (eds) CRC handbook of pest management in agriculture, Volume 1, 2nd edn. PL CRC Press, Inc., Boca Raton
-
Pimentel D et al (1992) Environmental and economic costs of pesticide use. BioScience 42:750–760
-
Popp J (2011) Cost-benefit analysis of crop protection measures. Journal of Consumer Protection and Food Safety 6(Supplement 1):105–112. doi:10.1007/s00003-011-0677-4, Springer, May 2011
-
REBECA (2007) Balancing the Benefits and Costs of Regulating Biological Plant Protection Products, WS 6 Synthesis, Deliverable No 25, Regulation of Biological Control Agents (REBECA)
-
Settle W, Garba MH (2011) The West African regional integrated production and pest management (IPPM) programme, a case study. UN Food and Agriculture Organization, Rome
-
Syngenta (2010) The MARGINS Project. Managing agricultural runoff generation into surface water. Syngenta Stewardship & Sustainable Agriculture, Basel
-
USDA (2010) Commodity costs and returns: U.S. and regional cost and return data. http://www.ers.usda.gov./data/costsandreturns (accessed 20 September 2010)
-
van den Bosch R (1978) The pesticide conspiracy. University California Press, Berkeley
-
Waage J (1997) Global developments in biological control and the implications for Europe. EPPO Bull 27:5–13
-
Yudelman M et al (1998) Pest management and food production: looking to the future. Food, agriculture, and the environment discussion paper 25. International Food Policy Research Institute, Washington, DC
-
Zadoks JC, Schein RD (1979) Epidemiology and plant disease management. Oxford University Press, Oxford
-
Zilberman D et al (1991) The economics of pesticide use and regulation. Science 253:518–522
Source: https://link.springer.com/article/10.1007/s13593-012-0105-x
0 Response to "The Pesticides Used in Farming Are Continually Re reviewed by the to Ensure Their Safety"
Post a Comment